The global blackberry (Rubus subgenus Rubus) industry has experienced rapid growth during the past 15 years, driven by increased consumer demand, advanced production methods, year-round product availability, and new cultivars. Despite the growing economic importance of blackberries and their excellent nutritional properties, few genomic resources exist to facilitate molecular breeding.
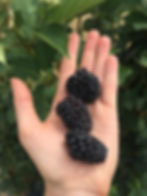
The application of molecular breeding in blackberry is a ‘thorny’ problem due to polyploidy, multisomic inheritance, and heterozygosity. While the red and black raspberries in subgenus Idaeobatus are diploids (2n = 2x = 14), cultivated blackberries in the subgenus Rubus are bred at the tetraploid (2n = 4x = 28) and higher order polyploid levels (Clark et al., 2007).
Today, we share the chromosome-length genome assembly for the diploid blackberry ‘Burbank Thornless’ (R. ulmifolius inermis, PI 554060), generated using plants donated by the USDA-ARS National Clonal Germplasm Repository in Corvallis, OR. The assembly was created using PacBio and Hi-C sequencing data. The PacBio data was assembled using FALCON software. The Falcon assembly was phased into haplotypes using FALCON-Unzip (see Chin, Peluso et al., 2016), with error correction on the phased assembly performed using Arrow. The Hi-C scaffolding was performed using the standard DNA Zoo workflow, based on in situ Hi-C (Rao, Huntley et al., 2014) prepared from fresh leaf samples. The tools used for Hi-C data processing included: Juicer (Durand, Shamim et al., 2016), 3D-DNA (Dudchenko et al., 2017) and Juicebox Assembly Tools (Dudchenko et al., 2018).
‘Burbank Thornless’ was chosen because it is believed to be closely related to ‘John Innes’, the source of the recessive gene for thornlessness in ‘Merton Thornless’, which has been used widely in fresh-market blackberry breeding programs (Coyner et al., 2005; Scott et al., 1957). Botanically speaking, it would be more accurate to describe this accession as prickle-free than thornless. Blackberries have prickles, outgrowths from epidermal tissue, instead of thorns or spines, which are connected to the vascular systems of the plant (more details on this here). Regardless of what they are called, anyone who has spent time picking berries in wild bramble patches can appreciate that picking from thornless cultivars is a much smoother experience!
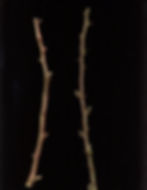
This work is part of a collaborative effort between the University of Arkansas, USDA-ARS, North Carolina State University, DNA Zoo, NIAB-EMR, Pairwise Plants, and the Wellcome Sanger Institute. Thanks to all involved: Erez Aiden, Rishi Aryal, Hamid Ashrafi, Nahla Bassil, Mario Caccamo, Brian Crawford, Michael Dossett, Olga Dudchenko, Felicidad Fernandez-Fernandez, Gina Fernandez, Jodi Humann, Sook Jung, Dorrie Main, Dan Mead, Cherie Ochsenfeld, Gina Pham, Melanie Pham, Tom Poorten, Dan Sargent, Aabid Shariff, Margaret Worthington, Xiaoyu Zhang. Find out more on the Genome Database for Rosaceae (GDR) webpage dedicated to the 'Burbank Thornless' blackberry genome assembly, here!
See below the whole-genome alignment plots that compare the genomes of the Burbank Thornless (R. ulmifolius) to the black raspberry (R. occidentalis V. 3, VanBuren et al., 2018) and woodland strawberry (Fragaria vesca V. 4, Edger et al., 2017). All three genomes are highly collinear. Inversions on chromosomes 1 and 7 were found between woodland strawberry and both Rubus species. Interestingly, the inversions between R. occidentalis and F. vesca previously documented on chromosomes 4 and 6 were not seen in the new assembly of Burbank thornless. These inversions likely represent errors in the chromosome-scale assembly of R. occidentalis.
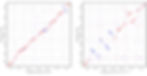
Citations:
Clark, J.R., Stafne, E.T., Hall, H.K., Region, N., and Finn, C.E. (2007). Blackberry breeding and genetics. Plant Breed. Rev. 29, 19–144.
Coyner, M.A., Skirvin, R.M., Norton, M.A., and Otterbacher, A.G. (2005). Thornlessness in blackberries: a review. Small Fruits Rev. 4, 83–106.
Edger, P.P., VanBuren, R., Colle, M., Poorten, T.J., Wai, C.M., Niederhuth, C.E., Alger, E.I., Ou, S., Acharya, C.B., Wang, J., et al. (2017). Single-molecule sequencing and optical mapping yields an improved genome of woodland strawberry (Fragaria vesca) with chromosome-scale contiguity. Gigascience 7, 1–7.
Scott, D.H., Darrrow G.M., and Ink D.P. (1957). ‘Merton Thornless’ as a parent in breeding thornless blackberries. Proc. Amer. Soc. Hort. Sci. 69,268-277.
VanBuren, R., Wai, C.M., Colle, M., Wang, J., Sullivan, S., Bushakra, J.M., Liachko, I., Vining, K.J., Dossett, M., Finn, C.E., et al. (2018). A near complete, chromosome-scale assembly of the black raspberry (Rubus occidentalis) genome. Gigascience 7, 1–9.