The Leadbeater's possum (Gymnobelideus leadbeateri) was named in 1867 after John Leadbeater, the then taxidermist at the Museum Victoria [1]. (They also go by the common name of fairy possum [2].) These cute little fairies which are just 33 cm (13 inches), tail included in body length, are rarely seen being nocturnal, fast-moving, and living in tree hollows of some of the tallest forest trees in the world [3]. They live in small family colonies of up to 12 individuals and mate twice per year, with a maximum of two joeys being born to each monogamous breeding pair in colony [4].

The Leadbeater’s possums belongs to the Petauridae family together with the gliding possums. In contrast to other members of the family, Leadbeater’s possums do not glide, and are thought to represent an ancestral form that evolved about 20 million years ago [5].
The State of Victoria, Australia, made the Leadbeater's possum its faunal emblem on 2 March 1971 [6], and since then this emblematic species has almost gone extinct! It is now listed as critically endangered, largely restricted to small pockets of alpine ash, mountain ash, and snow gum forests in the Central Highlands of Victoria, Australia, north-east of Melbourne, with a single isolated population in lowland floodplain forest [7, 8, 9]. In the highlands, the February 2009 Black Saturday bushfires destroyed massive part of the reserve system of Leadbeater's possums' habitat, and the wild population is thought to have been drastically reduced in size.
The availability of suitable habitat is critical for saving the species from looming extinction. Intensive population recovery measures, including translocation, will be required to save the last lowland population. The loss of hollow-bearing trees is the possums' biggest threat in highland habitats, along with bushfire. Suitable hollows can take 190 years to develop in living trees, and old trees with suitable hollows have decreased due to logging and bushfires in the wild over the last three decades of the 20th century [10]. The animal’s vulnerability to fire makes climate change a severe danger.
To support ongoing conservation efforts led by Zoos Victoria, DNA Zoo has been working with Paul Sunnucks and Alexandra Pavlova at Monash University to get a chromosome-length assembly genome for a female belonging to the sole remaining population of fewer than 30 individuals of lowland Leadbeater’s possum, which experience harmful effects of inbreeding [11].
The chromosome-length assembly we share today is based on the draft assembly available on NCBI was generated by Han Ming Gan, Stella Loke and Yin Peng Lee of Deakin Genomics Centre, and the Monash University team, with funding from Zoos Victoria and Australian Research Council funded project LP160100482 (Gymnobelideus leadbeateri isolate B50252). The draft genome assembly was created using MaSuRCA v. 3.3.4 (Zimin et al. 2013), using Oxford Nanopore MinION reads polished with short-insert size Illumina NovaSeq reads.
The draft was scaffolded to 11 chromosomes with 250M Hi-C reads generated by DNA Zoo labs from a liver sample from the same isolate, obtained from Leanne Wicker and Dan Harley (Zoos Victoria), using 3D-DNA (Dudchenko et al., 2017) and Juicebox Assembly Tools (Dudchenko et al., 2018). See our Methods page for more details!
The Hi-C work was supported by resources provided by DNA Zoo Australia, Faculty of Science, The University of Western Australia (UWA), DNA Zoo, Zoos Victoria and Monash University, with additional computational resources and support from the Pawsey Supercomputing Centre with funding from the Australian Government and the Government of Western Australia.
See below how the chromosomes from the new Leadbeater's possum genome assembly related to those of another notable Australian mammal from our collection, the tammar wallaby. That's about 55MY of evolution separating the species [12]. Check out the assembly page for the $1K tammar wallaby genome assembly here!
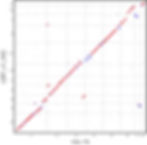
The following people contributed to the Hi-C chromosome-length upgrade of the project: Erez Aiden, Olga Dudchenko, David Weisz, Ruqayya Khan & Parwinder Kaur.
Blog by: Parwinder Kaur and Olga Dudchenko
Citations
Ruan, J. and Li, H. (2019) Fast and accurate long-read assembly with wtdbg2. Nat Methods doi:10.1038/s41592-019-0669-3
Dudchenko, O., Batra, S.S., Omer, A.D., Nyquist, S.K., Hoeger, M., Durand, N.C., Shamim, M.S., Machol, I., Lander, E.S., Aiden, A.P., Aiden, E.L., 2017. De novo assembly of the Aedes aegypti genome using Hi-C yields chromosome-length scaffolds. Science 356, 92–95. https://doi.org/10.1126/science.aal3327.
Dudchenko, O., Shamim, M.S., Batra, S., Durand, N.C., Musial, N.T., Mostofa, R., Pham, M., Hilaire, B.G.S., Yao, W., Stamenova, E., Hoeger, M., Nyquist, S.K., Korchina, V., Pletch, K., Flanagan, J.P., Tomaszewicz, A., McAloose, D., Estrada, C.P., Novak, B.J., Omer, A.D., Aiden, E.L., 2018. The Juicebox Assembly Tools module facilitates de novo assembly of mammalian genomes with chromosome-length scaffolds for under $1000. bioRxiv 254797. https://doi.org/10.1101/254797.
Durand, Shamim et al. “Juicer Provides a One-Click System for Analyzing Loop-Resolution Hi-C Experiments.” Cell Systems 3.1 (2016): 95–98.
James T. Robinson, Douglass Turner, Neva C. Durand, Helga Thorvaldsdóttir, Jill P. Mesirov, Erez Lieberman Aiden, Juicebox.js Provides a Cloud-Based Visualization System for Hi-C Data, Cell Systems, Volume 6, Issue 2, 2018